We have recently performed numerical simulations of star formation using
a Smoothed Particle Hydrodynamics (SPH) code that combines particle splitting
(Kitsionas & Whitworth 2002) and sink particles (Bate, Bonnell & Price 1995).
Our SPH code satisfies the Jeans condition (Truelove et al. 1997; Bate &
Burkert 1998) at all times by invoking on-the-fly particle splitting.
We have investigated the phenomenology of star formation triggered by
low-velocity collisions between low-mass molecular clumps. Such clump-clump
collisions are produced during larger scale interactions between
colliding gas flows, which constitute a frequent phenomenon in the turbulent
ISM.
In our simulations clumps are modelled as stable truncated (non-singular)
isothermal, i.e. Bonnor-Ebert, spheres. Collisions are characterised by
M (clump mass), b (offset parameter, i.e. ratio of
impact parameter to clump radius), and M (Mach Number,
i.e. ratio of collision velocity to effective post-shock sound speed).
The gas subscribes to a barotropic equation of state, which is intended to
capture (i) the scaling of pre-collision internal velocity dispersion with
clump mass, (ii) post-shock radiative cooling, and (iii) adiabatic heating
in optically thick protostellar fragments.
Fig. 1 shows the large-scale evolution of such clump-clump collisions: a
shock-compressed layer forms at the collision interface; this layer fragments
into filaments, which then break up into protostars.
An example of the small scale evolution of such a collision is shown in Fig. 2.
Two protostars fragment along the two ends of a tumbling filament (a third
protostar can be seen at the top of Fig. 2a being sheared out of the area
depicted by this panel). The protostars are captured into a binary as they
approach each other along the tumbling filament (Fig. 2b).
They form a tight binary (final separation <40 AU) attended by a large,
massive circumbinary disc (Figs. 2c and 2d). The disc fragments as it interacts
with material that gets accreted on it from the filament.
At the end of the simulation (Figs. 2e and 2f), a small group of 10
protostellar objects has formed: the binary (each component of ~1.5 solar
masses) and 8 low-mass objects, roughly half of which are of sub-stellar mass.
Only the binary is still heavily accreting. The other objects have been
ejected from the system (at moderate speeds), each after having experienced a close
encounter with the binary.
With SPH simulations combining particle splitting and sink particles we are
able to study the small-scale evolution of star-disc interactions by employing
only a moderate amount of computing resources: use of particle splitting
provides an economy in CPU ~90% and in memory ~75% (compared to simulations
without particle splitting which evolve at the same effective resolution).
We have therefore been able to follow disc fragmentation as a result of
star-disc interactions and/or accretion from a filament at a resolution
similar to that of Bate, Bonnell & Bromm (2003), by employing a significantly lower
amount of computing resources. Given that we can resolve the formation of structures down to ~20 AU
only, these results highlight the importance of star-disc
interactions for the formation of small-N clusters as well as for the
formation of close binaries and brown dwarves.
Bate M. R., Burkert A., 1997, MNRAS, 288, 1060
Bate M. R., Bonnell I. A., Price N. M., 1995, MNRAS, 277, 362
Bate M. R., Bonnell I. A., Bromm V., 2003, MNRAS, 339, 577
Kitsionas S., Whitworth A. P., 2002, MNRAS, 330, 129
Kitsionas S., Whitworth A. P.,
2007, MNRAS, in press
(astro-ph/0703177)
Truelove K. J., Klein R. I., McKee C. F., Holliman J. H.,
Howell L. H., Greenough J. A., 1997, ApJ, 489, L179
|
|
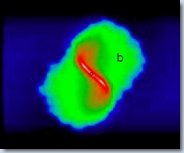
Fig.1 - Large scale evolution of one of our simulations, in particular a simulation
with M = 10 solar masses, b = 0.2, and
M = 10.
a. Initial conditions. The clumps move along the
x-axis (at 1 km/s each) and the offset, b, is taken along the y-axis.
Column density plots viewed along the z-axis; Delta x = 0.92 pc,
Delta y = 0.56 pc.
b. Same as a. Final state. A
shock-compressed layer forms at the collision interface; this layer fragments
into filaments, which then break up into protostars. Fig. 2 provides a zoom in
the area enclosed by the black rectangular.
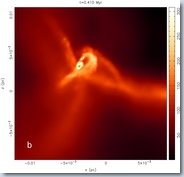
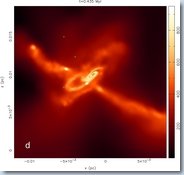
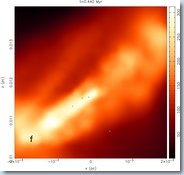
Fig. 2 - Small scale evolution of the simulation with M = 10 solar
masses, b = 0.2, and M = 10 (zooming in the area enclosed
by the black rectangular in Fig. 1b). All panels show column density along the
y-axis with Delta x = Delta z = 0.02 pc. The time of the snapshot is given at
the top of each panel. The colour bar on the right of each panel gives the
variation of column density in units of g/cm². Sink particles are
shown with green solid circles (note that the symbol used for the sinks is
larger than the actual sink radius at the scale of these plots, i.e. ~20 AU).
a. The result of filament fragmentation.
b. The two protostars are captured into a binary as they
approach each other along the tumbling filament.
c. and d.They form a tight binary (final separation <40 AU) attended by
a large, massive circumbinary disc. The disc fragments as it interacts
with material that gets accreted on it from the filament.
e. At the end of the simulation, a small group of 10
protostellar objects has formed.
f. Same as e. Zoom in the area around the binary and its
circumbinary disc (Delta x = Delta z = 0.004 pc).
|
Contact
Dr Spyridon Kitsionas
Astrophysikalisches Institut Potsdam
An der Sternwarte 16
D-14482 Potsdam
(0331) 7499 316
[Star formation program]
[AIP home page]
|
|